|
 |
 |
 |
 |
 |
 |
|
|
Scintillator
Properties Afterglow
Neutron Detection Radiation Damage Emission Spectra |
|
Temperature
Influence on Scintillation Response
Which Scintillator For Your Application? |
|
Scintillator Properties
A large number of different scintillation crystals exist for a variety of applications.
Some important characteristics of scintillators are:
Density and Atomic number
It is clear that for an efficient detection of g-rays, a material with a high density and high Z is
required (see above). Inorganic scintillation crystals meet the requirements of stopping
power and optical transparency, their densities ranging from roughly 3 to 9 g/cm3
makes them very suitable to absorb penetrating radiation (g-rays). Materials with high Z-values are used for g-ray spectroscopy at high energies (>1 MeV).
BACK TO TOP
Light output
Since photoelectron statistics (or electron-hole pair statistics) plays a key role in
the accurate determination of the energy of the radiation, the use of scintillation
materials with a high light output is preferred for all spectroscopic applications.
The scintillator emission wavelength should be matched to the sensitivity of the light
detection device that is used (PMT of photodiode).
BACK TO TOP
Decay time
Scintillation light pulses (flashes) are usually characterized by a fast increase of
the intensity in time (pulse rise time) followed by an exponential decrease. The decay
time of a scintillator is defined by the time after which the intensity of the light
pulse has returned to 1/e of its maximum value. Most scintillators are characterized by
more than one decay time and usually, the effective average decay time is mentioned. The
decay time is of importance for fast counting and/or timing applications.
Mechanical and optical properties
The most important scintillation material NaI(Tl) is hygroscopic and is only used in
hermetically sealed metal containers to preserve its properties. All water soluble
scintillation materials should be packaged in such a way that they are not attacked by
moisture. Some scintillation crystals may easily crack or cleave under mechanical pressure
whereas others, like CsI, are plastic and only will deform. In table 3.1 below,
the most important aspects of commonly used scintillation materials are listed. The list
is not exhaustive and new materials are developed regularly
Each scintillation crystal has its own specific application. For high resolution g-ray spectroscopy, NaI(Tl), or CsI(Na) (high light
output) are normally used. For high energy physics applications, the use of bismuth
germanate Bi4Ge3O12 (BGO) crystals (high density and Z)
improves the lateral confinement of the shower. For the detection of b-particles, CaF2(Eu) can be used instead of plastic
scintillators (higher density).
Below a short description of the most commonly used scintillators is presented. |
|
|
|
BACK TO TOP |
|
|
MATERIAL |
DENSITY [g/cm3] |
EMISSION MAXIMUM [nm] |
DECAY CONSTANT (1) |
REFRACTIVE INDEX (2) |
CONVERSION EFFICIENCY (3) |
HYGRO- SCOPIC |
NaI(Tl) |
3.67 |
415 |
0.23 ms |
1.85 |
100 |
yes |
CsI(Tl) |
4.51 |
550 |
0.6/3.4 ms |
1.79 |
45 |
no |
CsI(Na) |
4.51 |
420 |
0.63 ms |
1.84 |
85 |
slightly |
CsI (undoped) |
4.51 |
315 |
16 ns |
1.95 |
4 - 6 |
no |
CaF2 (Eu) |
3.18 |
435 |
0.84 ms |
1.47 |
50 |
no |
6LiI (Eu) |
4.08 |
470 |
1.4 ms |
1.96 |
35 |
yes |
6Li - glass |
2.6 |
390 - 430 |
60 ns |
1.56 |
4 - 6 |
no |
CsF |
4.64 |
390 |
3 - 5 ns |
1.48 |
5 - 7 |
yes |
BaF2 |
4.88 |
315 220 |
0.63 ms
0.8 ns |
1.50 1.54 |
16 5 |
no |
YAP (Ce) |
5.55 |
350 |
27 ns |
1.94 |
35 - 40 |
no |
GSO (Ce) |
6.71 |
440 |
30 - 60 ns |
1.85 |
20 - 25 |
no |
BGO |
7.13 |
480 |
0.3 ms |
2.15 |
15 - 20 |
no |
CdWO4 |
7.90 |
470 / 540 |
20 / 5 ms |
2.3 |
25 - 30 |
no |
Plastics |
1.03 |
375 - 600 |
1 - 3 ms |
1.58 |
25 - 30 |
no |
|
 |
 |
 |
 |
 |
 |
 |
 |
 |
 |
 |
 |
 |
 |
 |
 |
(1)
EFFECTIVE AGERAGE DECAY TIME FOR g-RAYS.
(2) AT THE WAVELENGTH OF THE
EMISSION MAXIMUM.
(3) RELATIVE SCINTILLATION
SIGNAL AT ROOM TEMPERATURE FOR g-RAYS WHEN . |
|
|
COUPLED TO A PHOTOMULTIPLIER TUBE WITH A Bi-ALKALI PHOTOCATHODE |
|
|
BACK TO TOP |
|
|
Afterglow
To
detect fast changes in transmitted intensity of X-ray beams, as e.g. in CT scanners or
luggage X-ray detectors, crystals are required exhibiting extremely low afterglow.
Afterglow is defined as the fraction of scintillation light still present for a certain
time after the X-ray excitation stops. Afterglow originates from the presence of
millisecond to even hour long decay time components. Afterglow in most halide
scintillation crystals can be as high as a few percent after 3 ms. The long duration
afterglow in e.g. CsI(Tl) can be a problem for many applications. Afterglow in halides is
believed to be intrinsic and correlated to certain lattice defects. BGO and Cadmium
Tungstate (CdWO4) crystals are examples of low afterglow scintillation
materials. |
|
|
|
BACK TO TOP |
|
|
Neutron Detection
Neutrons
do not produce ionization directly in scintillation crystals, but can be detected through
their interaction with the nuclei of a suitable element. In a 6 LiI(Eu)
scintillation crystal for example, neutrons interact with 6Li nuclei to produce
an alpha particle and a triton (tritium nucleus), which both produce scintillation light
that can be detected. For this purpose, also enriched 6Li containing glasses
can be used, doped with Ce as activator. |
|
|
|
BACK TO TOP |
|
|
Radiation Damage
Radiation damage is
defined as the change in scintillation characteristics caused by prolonged exposure to
intense radiation. This damage manifests itself by a decrease of the optical transmission
of a crystal which causes a decrease in pulse height and deterioration of the energy
resolution of the detector. Radiation damage other than radio-activation is usually
partially reversible; i.e. the absorption bands disappear slowly in time. In general,
doped alkali halide scintillators such as NaI(Tl) and CsI(Tl) are rather susceptible to
radiation damage. All known scintillation materials show more or less damage when exposed
to large radiation doses. The effects usually can only be observed clearly in thick (>
5 cm) crystals. A material is usually called radiation hard if no measurable effects occur
at a dose of 10.000 Gray. Examples of radiation hard materials are CdWO4 and
GSO. |
|
|
|
BACK TO TOP |
|
|
Emission Spectra
of Scintillation Crystals
Each
scintillation material has a characteristic emission spectrum. The shape of this emission
spectrum is sometimes dependent on the type of excitation (photons / particles). This
emission spectrum is of importance when choosing the optimum readout device (PMT
/photodiode) and the required window material. Fig. 3.1 and 3.2 show the emission spectrum of some common
scintillation materials. |
|
|
|
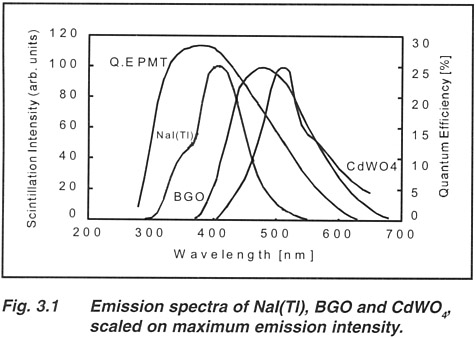 |
|
|
|
BACK TO TOP |
|
|
Temperature
Influence on the Scintillation Response
The
light output (number of photons per MeV gamma) of most scintillators is a function of
temperature. This is caused by the fact that in scintillation crystals, radiative
transitions, responsible for the production of scintillation light. compete with
non-radiative transitions (no light production). In most scintillation crystals, the light
output is quenched (decreased) at higher temperatures. An example of the contrary is the
fast component of BaF2 of which the emission intensity is essentially
temperature independent.. |
|
|
|
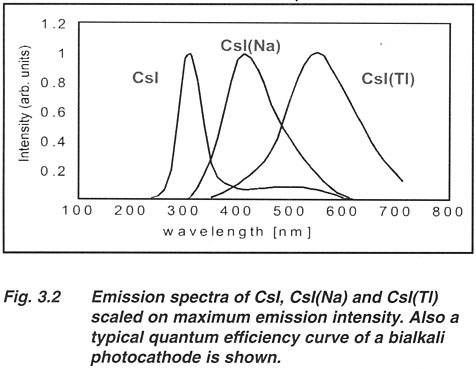 |
|
|
The scintillation process usually involves as
well production, transport and
quenching centers. Competition between these three processes each behaving differently
with temperature, causes a complex temperature dependence of the scintillation light
output. Fig. 3.3 shows the temperature dependence of some common
scintillation crystals. For
applications such as oil well logging and space research, where it is very difficult to
control the temperature, this dependence should be taken into account. The doped
scintillators NaI(Tl), CsI(Tl) and CsI(Na) show a distinct maximum in intensity whereas
many undoped scintillators such as BGO show an increase in intensity with decreasing
temperature.
From Fig. 3.3
it can be seen that at above 120 oC,
NaI(Tl) has the highest light output followed by CsI(Na) and CsI(Tl). For lower
temperatures CsI(Na) is a good alternative to NaI(Tl) because it has better mechanical
characteristics |
|
|
|
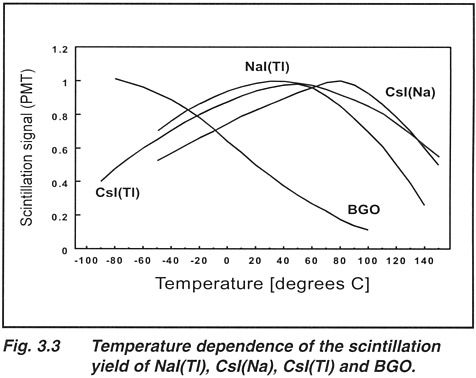 |
|
|
|
BACK TO TOP |
|
|
Which Scintillator
For Your Application?
When we
observe Table 3.1
carefully, it is
clear that none of presently known scintillation crystals possesses all the above
mentioned (ideal) characteristics such as high density, fast decay etc. The choice of a
certain scintillation crystal in a radiation detector depends strongly on the application.
Questions such as :
- What is the energy of the radiation to
measure ?
- What is the expected count rate ?
- What are the experimental conditions
(temperature, shock)?
are very important in this respect to determine the optimum choice.
Table 3.2 presents
an overview of the most commonly used scintillation materials with some of their specific
applications. |
|
|
MATERIAL |
IMPORTANT PROPERTIES |
MAJOR APPLICATIONS |
NaI(Tl) |
Very high light output, good energy resolution |
General scintillation counting, health physics, environmental monitoring,
high temperature use |
CsI(Tl) |
Noon-hygroscopic, rugged, long wavelength emission |
Particle and high energy physics, general radiation detection, photodiode
readout, phoswiches |
CsI(Na) |
High light output, rugged |
Geophysical, general radiation detection |
CsI(undoped) |
Fast, non-hygroscopic, radiation hard, low light output |
Physics (calorimetry) |
CaF2(Eu) |
Low Z, high light outut |
b detection, a, b phoswiches |
6LiI(Eu) |
High neutron cross-section, high light output |
Thermal neutron detection and spectroscopy |
6Li - glass |
High neutron cross-section, non-hygroscopic |
Thermal neutron detection |
BaF2 |
Ultra-fast sub-ns UV emission |
Positron life time studies, physics research, fast timing |
YAP(Ce) |
High light output, low Z, fast |
MHz X-ray spectroscopy, synchrotron physics |
GSO(Ce) |
High density and Z, fast, radiation hard |
Physics research |
BGO |
High density and Z |
Particle physics, geophysical research, PET, anti-Compton spectrometers |
CdWO4 |
Very high density, low afterglow, radiation hard |
DC measurement of X-rays (high intensity), readout with photodiodes,
Computerized Tomography (CT) |
Plastics |
Fast, low density and Z, high light output |
Particle detection, beta detection |
|
 |
 |
|
Medical probe with fiber optics light guide
and built-in HV supply |
|
|
|